I. Chemical Foundations
Topic 8 Overview
Concepts
- Kinetic-molecular theory of gases
- Impact of gases on the environment
Skills
- Inter-relationships of the gas laws
- Experimental manipulation of gases
- Acid-base titration measurements
You should be familiar with the contents of this section before you go to case study.
Proteins: Proteins are the main building blocks in animal and human systems. Proteins are made up of amino acids. The structure of proteins can be very complex; they can contain primary, secondary, and tertiary structures in the same molecule. The amino group of one amino acid molecule is linked to the acid group of a second molecule of the same or different amino acid by peptide bonds. The final structure of the protein is determined by the way hydrogen bonds connect the polypeptide (multiple amino acids bonded together) chains.
Amino acids: Amino acids are referred to as the building blocks of proteins. They are small organic molecules that contain an amino group (-NH2) and a carboxylic group (-COOH) on an adjacent carbon. Only 20 different amino acids occur in normal animal life and all proteins are polymers of these amino acids. Only 10 of these amino acids are manufactured by the body. The remaining amino acids come from the food we eat. Since amino acids are chiral compounds, only one enantiomer (the L isomer) is used to form proteins.
Chiral Compounds: When a carbon atom has a different substituent at each of its four bonds, then that compound is termed chiral. The general formula for amino acids is
The term chiral means handedness.
These compounds are either right- or left-handed mirror images of one another and cannot be superimposed on each other.
Enantiomer: Enantiomers are the mirror images of a single chiral compound; enantiomers are not superimposable on each other. Their physical properties will be the same, but they are optically discernible and will behave differently in the presence of other chiral molecules.
Diastereomer: Diastereomers are chiral compounds with more than one chiral center that are not mirror images of one another. These pairs of compounds have neither identical physical nor identical optical properties.
Structure of Proteins
- Primary Structure The primary structure of a protein is the sequence of amino acids along the protein chain.
- Secondary Structure The secondary structure describes how segments of the protein chain are folded. Some common secondary structures are α–helix, β-sheet, β-turn, γ-turn, etc.
- Tertiary Structure The tertiary structure describes the overall shape of the molecule. Proteins are large molecules, often containing hundreds of amino acids and they bend and fold to fit a given space. For instance, myoglobin (see Fig. 10.1) is a globular protein.
- Quaternary Structure The quaternary structure is the assembly of individual molecules (monomers or subunits) into a larger complex.
Myoglobin: The structures of myoglobin and hemoglobin were amongst the first protein structures to be determined by x-ray analysis. Myoglobin is a monomeric polypeptide of 153 amino acid residues (primary structure). Most (118 out of 153) of the amino acids are used by the protein to form the α–helices of the protein’s secondary structure. Eight α–helical segments form a tertiary structure of the protein that is nearly spherical, with a diameter of about 30Å. A strand representation of the structure of horse heart myoglobin depicts the secondary and tertiary structure of the protein, is shown on Fig. 10.1. The helices of the protein encompass a prosthetic group, a heme group (shown as black balls and sticks). The heme group binds a single iron ion in its center. For efficient oxygen binding by the working protein, the iron ion must be in the +2 state.
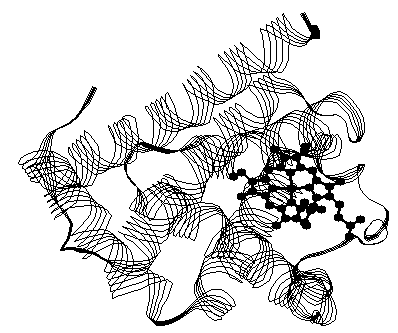
Within the hydrophobic pocket surrounding the heme group formed by the protein, the iron ion is coordinated to the four nitrogens of the porphyrin (heme), and to a fifth imidazole nitrogen of a histidine residue (87). The sixth coordination site of the iron is accessible to oxygen and other molecular ligands. (A ligand is an ion or a molecule that can bind to a metal ion or metal atom to form a complex.) In addition, a second histidine (residue 64) is located near the sixth coordination site and is thought to be important in stabilizing the oxygen during the bind-release cycle of the protein.
Iron-heme Complex The best known and most studied coordination compound is probably hemoglobin. Hemoglobin is important because it carries oxygen from the lungs to other parts of the body. Without this system, life could not exist as we know it. The iron•heme complex, shown in Fig. 10.2, is central to the function of myoglobin and hemoglobin. A very important property of this complex is the oxidation state of the bound iron ion. Although the iron•heme complex (ferroprotoporphyrin) can bind oxygen gas even when removed from the protein, the heme-bound iron(II) is rapidly oxidized to iron (III) without the protein. In the ferric state (Fe3+), the heme-bound iron is incapable of reversible oxygen binding. Even when the iron•heme complex is bound to the protein, the oxidation of the iron (from Fe2+ to Fe3+) is accompanied by loss of function. The structure of the protein assembly is thought to stabilize the ferrous (Fe2+) state and to enable reversible oxygen binding at the iron site.
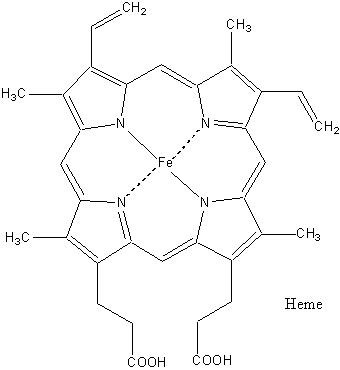
Although oxygen is the intrinsic ligand to normal hemoglobin function, the study of other ligands is also of interest. For instance, carbon monoxide poisoning is the leading cause of poisoning deaths in the U.S. Studies of carbon monoxide and oxygen binding equilibria to myoglobin and the related protein hemoglobin, enables the molecular-level understanding of the cause and treatment of carbon monoxide poisoning. Although the specific experimental details that scientists use to probe such equilibria depend on the exact nature of the ligand involved in the binding, a widely used strategy for investigations of this type involves adding various amounts of ligand to a known amount of protein and following the chemical binding spectroscopically.
Structure-Function Analysis of Proteins: One way to investigate the structure-function relationships that exist in proteins such as myoglobin is to compare the structure of proteins with similar function and structure. One such study by Perutz and Lehmann, looked at the primary sequence of vertebrate myoglobins and hemoglobins; one conclusion of the study was that 7 amino acid residues (7 out of ~153) were invariant in all species and appeared in identical positions in all normally functioning myoglobins. In those instances where, due to mutations, different amino acid residues are in these critical locations, the normal binding function is destroyed. Of these seven critical residues, most are located around the heme group.
Oxidation of Ferrohemoglobin: A Biological Example Ferrohemoglobin (Hb with 4 Fe2+) can easily be oxidized to ferrihemoglobin (Hb+ with 4 Fe3+) using suitable oxidizing agents. When the iron is present in the +3 oxidation state, the binding properties of the iron ion are modified; ferrihemoglobin binds ions such as OH– and F– rather than molecules such as O2.
The biological impact of this oxidation can be severe. It is the chemical basis of blue baby syndrome
(methemoglobinemia). If nitrate in the blood stream is reduced to nitrite, the ferrohemoglobin in the baby’s blood stream is oxidized to ferrihemoglobin. Once the hemoglobin is converted to ferrihemoglobin (also known as methemoglobin), the transport of oxygen effectively stops and the baby turns blue due to oxygen deprivation.
In contrast to the complex oxygen binding properties of ferrohemoglobin, in which the four subunits of the protein act in a cooperative manner, the binding of ligands by ferrihemoglobin may be simpler, because it is assumed that each subunit binds ligands independently. In other words, the overall equilibrium has a single equilibrium constant.