II. Chemical Foundations
You should be familiar with the contents of this section before you go to case study.
Oxidation: Oxidation is a process in which a substance loses one or more electrons. An example of oxidation is:
H2 → 2H+ + 2e–
Reduction: Reduction is a process in which a substance gains one or more electrons. An example of reduction is:
O2 + 4e– → 2O2-
Redox Half Reactions: Oxidation and reduction always occur together. An oxidizing agent must be present for an oxidation reaction to take place. The oxidizing agent is reduced in the process. Equations for this process can be written for each of these processes and then added together to reflect the entire reaction. For example:
Cu2+(aq) + 2e- ⇄ Cu(s)
Zn(s) ⇄ Zn2+(aq) + 2e–
Overall reaction: Cu2+(aq) + Zn(s) ⇄ Cu(s) + Zn2+(aq)
Electrochemical Cells: Electricity is produced by arranging equipment in such a way that electricity can be produced from spontaneous redox reactions. The classic electrical cell (a.k.a. voltaic or galvanic cell) is diagrammed in Fig. 12.3. The cell is formed by two half-cells connected by a salt-bridge. A voltmeter is wired to each half-cell to measure the developed voltage.
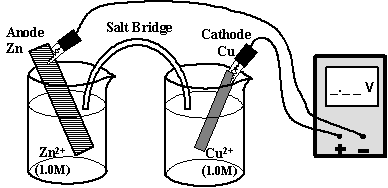
Concentration Cells: A concentration cell has the same substance on both sides of the cell (see Fig. 12.4) but the concentrations of the two solutions are different. Because the tendency for reduction increases with increased concentration, the more concentrated solution becomes more dilute and the more dilute solution becomes more concentrated setting up a cell potential. Eventually both solutions have the same concentration. Instead of mixing the solutions together, the cell uses the two solutions as half-cells and copper electrodes.
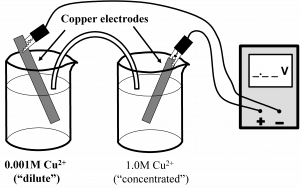
In the dilute cell (the anode, bold) copper metal is oxidized to form Cu2+ ions. The electrons liberated by the oxidation of copper metal flow through the wire to the cathode where Cu2+ ions are reduced. The net effect is to make the dilute solution more concentrated and the concentrated solution more dilute, until both solutions are the same concentration (0.5 M). The chemical reaction for this process can be written as:
Cu2+(aq, 1 M) + 2e– ⇄ Cu(s) cathode (+)
Cu(s) ⇄ Cu2+(aq) + 2e– anode (-)
Cu2+(aq, 1M) + Cu(s)⇄ Cu(s) + Cu2+(aq)
The bold face type refers to the dilute copper cell and the normal type refers to the half-cell with 1.0 M Cu2+.
If the concentration of the more concentrated solution is known, the concentration of the other cell can be calculated using the Nernst equation (see below). This is a very useful method for measuring very small quantities of ions.
Representation of an Electrochemical Cell: Rewriting the cell represented under “Redox Half-Reactions” using the conventional notation, the reaction at the anode is written to the left of the double lines in the center, and the cathode reaction is written to the right.
Zn(s) | Zn2+(aq) || Cu2+(aq) | Cu(s)
In this case the single line between Zn(s) and Zn2+(aq) represents the phase boundary in the anode portion of the cell, the single line between Cu2+(aq) and Cu(s) represents the phase boundary at the cathode; the double bars (||) between the cathode and the anode half reactions represent the salt bridge.
Standard Reduction Potential: Eºred is used to represent the voltage generated in a cell under standard conditions (P = 1 atm, T = 25ºC, and c = 1M) measured against a Standard Hydrogen Electrode.
Standard Hydrogen Electrode: Under standard conditions (P = 1 atm, T = 25ºC, and c = 1M H+), the reduction potential of H+ is defined as zero volts, according to the following half-cell reaction:
2H+ (1 M) + 2e– → H2 (1 atm)
Gibbs Free Energy: Since free energy (ΔG) is defined as the maximum amount of useful work that is done, that work when expressed as electrical energy (nFEcell) can be related to free energy as
ΔG = -nFEcell
Note that the negative sign indicates that the work is done on the surroundings of the cell.
Relationships between ΔGº, K, and Eºcell
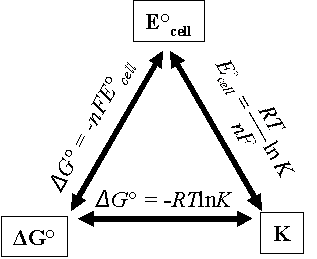
Units:
• Volt – the unit that measures the difference in electrical potential needed to move electrons.
• Coulomb – total electrical charge, which is equivalent to the number of moles of electrons times the Faraday constant (see next entry).
• Faraday – the electrical charge in 1 mole of electrons and is equivalent to 96,500 coulombs (C).
Cell Potential: The standard cell voltage (Eºcell) can be obtained from the standard reduction potentials of the two half-cells according to the equation
Eºcell = Eºred (cathode) – Eºred (anode)
The cell voltage Eºcell gives the voltage of an electrochemical cell in which the products and reactants are in the standard states (gases at 1 atm, solutes at 1 M, solids in their pure, most stable form, and temperature is 298 K). The cell voltage is also a measure of the spontaneity of a redox type reaction. In fact, relationships exist between the standard cell potential (Eºcell), and other measures of spontaneity such as the standard Gibbs free energy (ΔGº), and the equilibrium constant Keq, as given by
ΔGº = -nFEºcell = – RTln(Keq)
as shown in Fig. 11.3, where n is the number of moles of electrons transferred in the balanced half-reaction, F is Faraday constant (9.65 ×104 J/V•mol), R is the gas constant and T is the temperature (in Kelvin).
Nernst Equation: For conditions other than the standard conditions, the cell potential has been found to follow the mathematical form given by the Nernst equation
Ecell = Eºcell – (RT/nF) lnQ
where Q is the reaction quotient.
At room temperature (298 K), the Nernst equation is equal to
Ecell = Eºcell – (0.0257V/n) lnQ = Eºcell – (0.0592V/n) log10Q
(Note: 2.303 ln = log10)
When the reaction quotient is not equal to 1, the cell potential, Ecell, differs from the standard cell potential, Eºcell. The concentration dependence of the cell potential is found within the reaction quotient.
pH Measurement: Hydroquinone (C6H4(OH)2) and quinone (C6H4O2) react with each other to form a loosely bound complex known as quinhydrone. When quinhydrone is dissolved in solvent, it makes a solution that has an equal concentration of quinone and hydroquinone. If we consider the following reaction where quinone and hydroquinone appear on both sides of the chemical equation, the half-reactions and overall equation can be written as:
C6H4O2 + 2H+ + 2e– ⇄ C6H4(OH)2 cathode (+)
C6H4(OH)2 ⇄ C6H4O2+ 2H+ + 2e– anode (-)
C6H4(OH)2 + C6H4O2+ 2H+ ⇄ C6H4O2+ 2H+ + C6H4(OH)2
The bold face type refers to the chemicals in one half-cell at one concentration and the normal type refers to the same chemical species in the other half-cell at a different concentration. The pH can be measured using the reversible oxidation-reduction of hydroquinone to quinone. Since H+ appears in the chemical reaction, it also appears in the reaction quotient, yielding a dependence of the cell potential on the pH.
The Nernst equation for this cell can be written as
The concentrations of quinone and hydroquinone cancel in each case since they are present in equal concentrations, leaving a function that simply depends on the concentration of [H+]. This equation can be rewritten as,
E = 0.0592 V × (pH-pH) = 0.0592 V × ΔpH
If the system behaves ideally, a reference solution (a solution with a known pH) can be used to form one of the half cells and the pH of the unknown solution can be determined simply by constructing the appropriate electrochemical cell and measuring the cell potential. For non-ideal systems, a calibration curve can be constructed using several unknowns.
Batteries: A battery is either a single electrochemical cell or more generally a series of electrochemical cells designed to deliver a constant voltage at a constant rate. There are numerous types of batteries.
Type of Battery | Uses |
---|---|
Zinc-carbon Dry Cell | Flashlights, transistor radios |
Alkaline | general purpose |
Mercury | medicine, electronics |
Lead Storage | automobiles (rechargeable) |
Solid-state Lithium-ion | (rechargeable, high current) |
Nickel-cadmium (nicad) | (rechargeable) |
Nickel-metal hydride | (rechargeable, high current) |
The cathode of a battery is designed with a plus (+) sign and the anode with a minus (-) sign.Fuel Cells: For additional information on fuel cells, read http://www.howstuffworks.com/fuel-cell.htm.
Fuel cells are voltaic cells that can convert the energy produced by reacting hydrogen (or a hydrocarbon derivative) at the anode and oxygen at the cathode according to the following reactions
4e– + O2(g) + 2H2O(l) → 4OH–(aq) cathode (+)
2H2(g) + 4OH– → 4H2O(l) + 4e– anode (-)
2H2(g) + O2(g) → 2H2O(l)
Fuel cells differ from true batteries because they are not self-contained systems. They require a constant supply of hydrogen and oxygen.